Everything about
Microstereolithography and 3D Microfabrication
Everything about
Microstereolithography and 3D Microfabrication
Advancements in 3D Microfabrication: Microstereolithography Beyond Traditional MEMS Techniques
Microstereolithography in combination with photopolymers addresses current challenges of 3D microfabrication. It enables high-resolution, complex geometries and rapid prototyping.
Unlike traditional MEMS techniques, it offers flexibility, reduces material waste, and lowers production costs. The following sections shed light on modern microstereolithography and how it complements traditional MEMS techniques.
3D Microfabrication: Definition
3D microfabrication involves creating three-dimensional structures at microscale, typically from a few micrometres to millimetres. This technique includes various methods such as photolithography, electron beam lithography, laser lithography, and additive manufacturing processes such as microstereolithography.
Microstereolithography (Micro SLA) is an advanced 3D printing technique used to fabricate intricate microscale structures with high precision. It works by using a focused light source, typically a laser, to selectively cure and solidify layers of a photopolymer resin. This process is repeated layer by layer, allowing for the creation of complex three-dimensional objects.
These methods cater to different aspects of microfabrication, providing flexibility and precision in creating microscale components used in various applications, including microelectromechanical systems (MEMS).
Types of 3D Microfabrication Techniques
3D microfabrication techniques are used to create complex structures at the microscale, combining high precision and accuracy to meet the requirements of advanced applications in fields like electronics, optics, and biomedical engineering. These methods range from additive to subtractive manufacturing and rely on various energy sources to shape materials at the microscopic level. Below are some of the most common and widely-used techniques in 3D microfabrication.
Laser Lithography
Laser lithography is a versatile technique that uses a focused laser beam to pattern photosensitive materials. Unlike photolithography, laser lithography can be employed for both 2D and 3D microfabrication. By adjusting the focal plane of the laser, it’s possible to create structures at different depths, making it suitable for fabricating more complex geometries.
This method is widely used in industries such as optics and microelectronics, as well as for producing microfluidic devices. Laser lithography offers high precision and flexibility and is particularly beneficial for applications requiring complex microstructures or rapid prototyping.
Microstereolithography
Microstereolithography, or Micro SLA, is an advanced 3D microfabrication technique that allows for the creation of highly intricate 3D structures. It works by using a focused light source, typically a laser, to selectively cure liquid photopolymer resin in a layer-by-layer process. This technique enables the fabrication of highly detailed and complex geometries at the microscale, with resolutions as fine as a few micrometers.
Micro SLA is often used in industries that require high precision and custom designs, such as biomedical engineering, MEMS, and micro-optics. Its ability to build complex 3D structures with minimal material waste and high resolution makes it an ideal choice for rapid prototyping and production of functional microdevices.
Electron Beam Lithography
Electron Beam Lithography (EBL) is a high-resolution technique that uses a focused beam of electrons to draw custom patterns on a substrate coated with an electron-sensitive resist. Unlike photolithography, EBL does not require a mask and can achieve feature sizes down to a few nanometers, making it ideal for applications requiring extreme precision, such as quantum devices and nanoscale structures.
EBL offers flexibility in design but is a relatively slow and expensive process, often used for research and prototyping rather than mass production. It is particularly useful for fabricating complex patterns and devices at the nanoscale, where conventional photolithography falls short in resolution.
Photolithography
Photolithography transfers patterns onto a substrate using light. A photosensitive material (photoresist) is applied and exposed to UV light through a mask. The exposed areas either become soluble or remain insoluble, depending on the type of photoresist. This technique is widely used in microelectronics and MEMS for its high-resolution capabilities but is mainly suited for 2D structures.
What is MEMS?
MEMS, or Microelectromechanical Systems, are miniature devices that integrate mechanical and electrical components on a microscale. Typically ranging in size from a few micrometers to millimeters, MEMS technology combines elements such as sensors, actuators, microstructures, and microelectronics on a single chip or substrate. These systems are widely used in various industries, including automotive, healthcare, consumer electronics, and telecommunications, due to their ability to perform mechanical functions with high precision at a small scale.
MEMS devices are fabricated using processes similar to those used in semiconductor manufacturing, such as photolithography, etching, and deposition.
However, unlike traditional integrated circuits (ICs) that only process electrical signals, MEMS devices can sense, control, and actuate physical processes. This allows them to interact with their environment in real-time, making MEMS crucial for applications such as accelerometers, gyroscopes, pressure sensors, and microfluidic devices.
The flexibility of MEMS technology enables a wide range of applications, from everyday devices like smartphones and airbags to more complex systems in medical diagnostics, robotics, and aerospace. Its ability to combine electrical and mechanical functionalities on a small scale makes MEMS a versatile and powerful solution in modern technology.
Bulk Micromachining
Bulk micromachining is a process, where material is selectively removed from a bulk substrate, typically silicon, to create three-dimensional microstructures. This technique involves methods such as wet or dry etching to form cavities, channels, and through-holes. Bulk micromachining is essential for creating MEMS components, such as pressure sensors and accelerometers, that require precise and robust structural elements.
Surface Micromachining
Surface micromachining builds up microstructures on the surface of a substrate through sequential deposition and patterning of thin films. Unlike bulk micromachining, which etches into the substrate, surface micromachining forms structures layer by layer using materials such as polysilicon, silicon dioxide, and silicon nitride. This technique is widely used in creating MEMS devices, such as microactuators and micromirrors, where precise control over thin film dimensions and properties is essential.
LIGA (Lithographie, Galvanoformung, Abformung)
LIGA is a microfabrication technology used to create high-aspect-ratio microstructures with precise dimensions and structural integrity. The process involves three main steps:
1. Lithography to define the microstructure pattern using deep X-ray or UV lithography
2. Electroforming (Galvanoformung) to deposit metal into the patterned moulds
3. Moulding (Abformung) to replicate the structures in various materials
Silicon Micromachining
Silicon micromachining is a process for fabricating intricate microstructures on silicon wafers, commonly used in MEMS production. It enables the creation of precise microscale components such as sensors, actuators, and microfluidic devices, which are crucial for applications in automotive, aerospace, medical, and consumer electronics industries.
Challenges of MEMS and 3D Microfabrication
MEMS and 3D microfabrication still face several challenges in achieving optimal performance and efficiency. The following paragraphs outline the most important challenges.
Dimensional Accuracy
This challenge involves that fabricated components need to meet precise dimensional specifications and intricate geometries. Achieving high dimensional accuracy requires overcoming issues such as material shrinkage, process variability, surface roughness, and alignment errors during fabrication. Additionally, factors like thermal expansion and mechanical stresses can further affect dimensional stability.
Compatibility
Process compatibility ensures that various fabrication techniques, such as lithography, etching, deposition, and bonding, can be combined effectively to produce complex microscale structures with desired functionalities. Compatibility considerations include material compatibility, process compatibility (e.g., compatibility of temperature, chemical reactions), and compatibility of equipment and tooling.
Material Selection
The challenges of material selection in MEMS and 3D microfabrication involve identifying and utilising materials that can meet the stringent requirements of miniaturisation, functionality as well as environmental conditions. These challenges stem from the need to balance conflicting material properties such as mechanical strength, thermal stability, electrical conductivity, and biocompatibility while considering fabrication constraints and cost-effectiveness. Additionally, integrating multiple materials with disparate properties poses challenges in achieving compatibility and a reliable performance of microscale devices.
Reliability and Durability
Assuring reliability and durability becomes even more difficult with microscale devices and structures that are subject to varying environmental conditions. Challenges stem from factors such as material degradation, manufacturing defects and mechanical reliability, which can lead to device failure or performance degradation over time.
Miniaturisation vs. Functionality
The previous points lead to the last challenge: the balance of miniaturisation vs. functionality. This is crucial, as shrinking dimensions can lead to problems in maintaining functionality due to constraints in material properties, fabrication techniques, and integration of complex features, just to name a few.
Microstereolithography: A complement solution to MEMS
Microstereolithography can be a complementary solution to the aforementioned challenges. With microstereolithography, high precision and resolution are achievable, enabling the production of intricate microscale structures with unprecedented detail. Complex geometries, often difficult to achieve with conventional manufacturing methods, become feasible through this technology, which in turn unlocks new possibilities for MEMS designs.
Microstereolithography offers further advantages in terms of rapid prototyping and iterative design processes. Its ability to produce functional prototypes with minimal lead time facilitates faster iterations, which ultimately reduces time-to-market and development costs. Therefore, microstereolithography offers a great solution to enhance production processes as well as the quality of the final products.
How Microstereolithography Complements MEMS
Microstereolithography (Micro SLA) enhances MEMS (Microelectromechanical Systems) by enabling the fabrication of highly intricate, 3D microstructures that traditional MEMS techniques struggle to achieve. While MEMS fabrication techniques such as bulk and surface micromachining are well-suited for 2D and planar structures, Micro SLA excels in building complex, high-resolution 3D geometries.
This capability allows MEMS designers to integrate more advanced, non-planar structures, expanding the functionality of MEMS devices in fields like medical diagnostics, sensors, and microfluidics. By working alongside conventional MEMS processes, microstereolithography enables a more flexible, faster prototyping cycle and can overcome material and design limitations in existing MEMS technology.
Advantages of Microstereolithography for 3D Microfabrication
Microstereolithography offers several key advantages in 3D microfabrication:
High Precision and Resolution: Micro SLA achieves extremely fine resolutions down to a few micrometers, making it ideal for applications requiring detailed microstructures.
Complex Geometries: Unlike traditional 2D MEMS methods, Micro SLA builds fully three-dimensional structures, allowing for more complex and functional designs in fields like MEMS and micro-optics.
Material Efficiency: Micro SLA uses photopolymers and minimizes material waste, reducing production costs and enabling rapid prototyping with lower resource use.
Customizability: The technique allows for highly customizable designs and rapid iterations, which is especially valuable in research and development phases where design flexibility is crucial.
Integration with Existing MEMS: Micro SLA can complement existing MEMS processes, allowing for the creation of hybrid devices that integrate both additive and subtractive fabrication methods.
Applications of Microstereolithography in High-Precision 3D Printing
Microstereolithography is widely used in applications where high precision and intricate details are required:
Biomedical Devices: Micro SLA is used to create microfluidic devices, surgical tools, and custom implants that require fine detail and precision in both design and function.
MEMS: In MEMS, Micro SLA allows for the creation of more complex sensors, actuators, and microstructures, expanding the potential for advanced MEMS applications in industries like automotive, aerospace, and consumer electronics.
Optics and Micro-Optics: The technology is frequently used in the fabrication of micro-lenses and optical devices, where precise control over geometry and surface finish is essential.
Prototyping and Research: Micro SLA's ability to rapidly produce prototypes of intricate designs enables faster development cycles, making it ideal for research labs and industries focused on innovation.
RAHN: Your Partner for Advanced 3D Printing Solutions
RAHN is your trusted partner for cutting-edge 3D printing solutions that bring your next project to life. Experience unparalleled precision, versatility, and efficiency with our advanced materials for 3D printing resins tailored to your specific needs. Contact us today to find out more.
Your personal contact
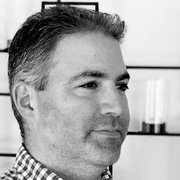
Christopher Cocklan
3D Printing Business Development Manager EnergyCuring
RAHN USA Corp.